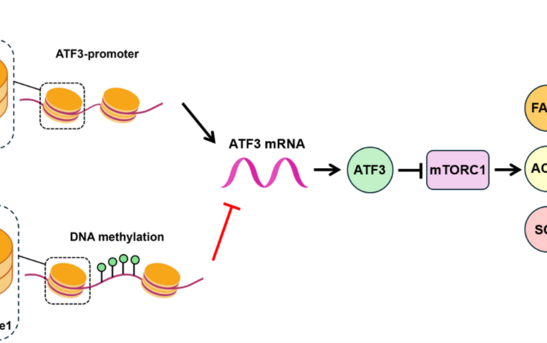
HKU Biologists Uncover How a Genetic Player Fuels Liver Cancer by Disrupting Fat Metabolism
Figure 1. Mechanism of how genetic player VPS72 impacts liver cancer. Too much VPS72 in cells activates the cancer-promoting pathway, which increases fat production and helps liver cancer grow. This study shows a new connection between fat buildup and liver cancer, suggesting new treatment possibilities. Image adapted from the respective paper at Advanced Science. Liver cancer is a lethal disease with limited treatment options for advanced stages. While risk factors such as viral hepatitis, alcohol use, and obesity increase the likelihood of hepatocellular carcinoma (HCC, the progression of liver cancer), scientists are racing to uncover the hidden biological mechanisms that fuel tumour growth. Recent research led by Professor Jiangwen ZHANG of the School of Biological Sciences at The University of Hong Kong (HKU) has uncovered how a key genetic culprit, VPS72, drives the development of liver cancer by disrupting fat metabolism and manipulating gene activity. The team’s findings have been published in Advanced Science. The Fat-Liver Cancer Connection The liver is the body’s metabolic powerhouse, responsible for balancing fat production and breakdown. In healthy individuals, this process is tightly controlled. However, in HCC, liver cells accumulate excessive fat droplets, fuelling tumours to grow uncontrollably. This fat buildup is largely controlled by a key cellular pathway known as mTORC1, a molecular switch that activates fat-producing genes, and by SREBP proteins, which act as master regulators that directly control enzymes involved in fat synthesis. In cancer cells, mTORC1 becomes hyperactive, ramping up fat production and creating a vicious cycle that promotes tumour growth. The Role of Genetic Driver VPS72 in Liver Cancer Development Professor Zhang and his team discovered that VPS72, a gene that modifies how DNA is packaged inside cells, is overactive and abnormally amplified (i.e., present in extra copies) in over 50% of HCC patients. This amplification is linked to poorer survival rates, suggesting that this genetic player plays a central role in cancer progression. Here is how the mechanism works: DNA Packaging: VPS72 helps attach specific proteins to DNA, which affects whether certain genes are turned on or off. Suppressing Protective Genes: VPS72 adds chemical tags to the promoter of gene ATF3, which normally helps prevent cancer growth. This tagging shuts down the production of ATF3, leading to the overactivity of a cancer-promoting pathway called mTORC1. Increasing Fat Production: When mTORC1 is overactive, it boosts proteins that increase the production of fats. This results in cancer cells being flooded with fats, providing them with energy and materials needed to grow rapidly. In short, this genetic player acts like a rogue conductor: it silences protective genes, promotes the cancer pathway and forces liver cells to overproduce fat —a perfect storm for cancer. A New Target for Liver Cancer Treatment These findings offer new hope for targeted therapies. One approach is to design drugs that block VPS72's interaction with H2A.Z proteins, potentially stopping the DNA changes that lead to cancer. Another strategy involves using existing drugs that inhibit mTORC1, a pathway already targeted in other cancers, which could be repurposed for liver cancer patients with increased VPS72 activity. By focusing on VPS72 and the pathways it affects, Professor Zhang’s team hopes to stop cancer from progressing. “Our research shows that the gene VPS72 plays two key roles in liver cancer (HCC): it affects both how genes are controlled and how fat metabolism goes wrong. The findings help explain how liver cancer develops and suggest new ways to treat cancers driven by abnormal fat metabolism,” said Professor Jiangwen Zhang, corresponding author of the study. This research uncovers a critical link between genetic regulation, fat metabolism, and liver cancer. By targeting the genetic player VPS72 and its cancer-promoting pathway, scientists hope to open the door to more precise and effective treatments—potentially turning off a key fuel supply that liver tumours depend on. The full research paper can be accessed at: https://advanced.onlinelibrary.wiley.com/doi/10.1002/advs.202411368 Figure 2. Professor Jiangwen ZHANG (second from the left) from HKU School of Biological Sciences and his research team.
NEWS DETAIL
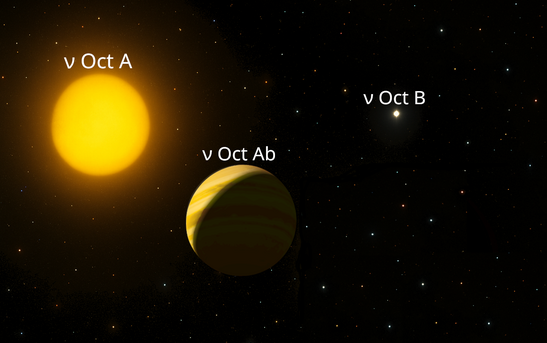
HKU Astrophysicists Lead Research Team to Uncover the Role of Binary Star Evolution in the Origin of a Retrograde Planet
Most stars in the Universe exist in binary or multiple star systems, where the presence of close-in companion stars in such systems can adversely influence the formation and orbital stability of planets around one of the stars. An international team of astrophysicists led by Professor Man Hoi LEE from the Department of Earth Sciences and the Department of Physics at The University of Hong Kong (HKU) and Mr Ho Wan CHENG, an MPhil student in his team, has confirmed the existence of a planet in an unprecedented retrograde orbit (moving in the opposite direction to the binary’s orbit) in the nu Octantis binary star system and revealed the role of binary star evolution in the origin of this planet. The findings have been published in the journal Nature. nu Octantis is a tight binary star system comprising a primary subgiant star, nu Oct A, with about 1.6 times the mass of the Sun, and a secondary star, nu Oct B, with about half the mass of the Sun. The two stars orbit each other with a period of 1,050 days. An additional periodic signal in the radial velocity observations (measurements of how a star moves towards or away from us) of this system was first reported by Dr David RAMM, a co-author of this new paper, during his PhD studies at the University of Canterbury, New Zealand, in 2004. This signal was consistent with the presence of a Jovian planet of about twice the mass of Jupiter orbiting around the primary star, nu Oct A, with a period of about 400 days. However, the existence of this planet has been controversial because its orbit would be so wide that it could only remain stable if it were retrograde and moved in the opposite direction to the orbit of the binary. There were no observational precedents for such a planet and strong theoretical grounds against its formation. To settle the debate, the research team obtained new high-precision radial velocity observations using the European Southern Observatory (ESO)’s HARPS spectrograph, which confirmed the existence of the planet signal. ‘We performed an analysis of the new and archival radial velocity data spanning 18 years and found stable fits that require the planetary orbit to be retrograde and nearly in the same plane as the binary orbit,” said Mr Ho Wan CHENG, the first author of the paper. Another key focus of the new study was the determination of the nature of the secondary star nu Oct B. The mass of nu Oct B suggests that it could be either a low-mass main-sequence star or a white dwarf. All stars spend most of their lives on the main sequence, generating energy through nuclear fusion of hydrogen to helium in their core. After a star has exhausted its nuclear fuel, its core collapses into a stellar remnant, which would be a white dwarf if the star’s initial mass is less than several times that of the Sun. A white dwarf has a mass comparable to that of the Sun packed in an Earth-sized volume. To identify which type of star nu Oct B is, the research team used the adaptive optics imaging instrument SPHERE at ESO’s Very Large Telescope to observe the system. The fact that nu Oct B was not detected in these observations indicated that it must be a very faint white dwarf. This suggests that the binary system has evolved significantly since its formation, as nu Oct B has already ejected most of its mass and entered the final stage of its stellar evolution. The research team looked into the possible primordial configurations of the binary — that is, the initial masses of the two stars and the initial orbit of the binary. ‘We found that the system is about 2.9 billion years old and that nu Oct B was initially about 2.4 times the mass of the Sun and evolved to a white dwarf about 2 billion years ago,’ said Cheng. ‘Our analysis showed that the planet could not have formed around nu Oct A at the same time as the stars.’ The discovery that nu Oct B is a white dwarf opens new possibilities for how the retrograde planet may have originated. ‘When nu Oct B evolved into a white dwarf about 2 billion years ago, the planet could have formed in a retrograde disc of material around nu Oct A accreted from the mass ejected by nu Oct B, or it could be captured from a prograde orbit around the binary into a retrograde orbit around nu Oct A,’ explained Professor Man Hoi LEE. ‘We might be witnessing the first compelling case of a second-generation planet; either captured, or formed from material expelled by nu Oct B, which lost more than 75% of its primordial mass to become a white dwarf, ’ added Dr Trifon TRIFONOV of Zentrum für Astronomie der Universität Heidelberg in Germany and Sofia University St. Kliment Ohridski in Bulgaria and a co-author of the paper. ‘The key to this exciting discovery was the use of several complementary methods to characterise the system in its entirety,’ said PD Dr Sabine REFFERT of Zentrum für Astronomie der Universität Heidelberg and another co-author of the paper. As astronomers continue to search for planets in different environments, this study highlights that planets in tight binary systems with evolved stellar components could offer unique insights into the formation and evolution of planets. This research utilises two facilities operated by the European Southern Observatory (ESO), specifically the High Accuracy Radial Velocity Planet Searcher (HARPS) spectrograph at the ESO's 3.6-metre La Silla telescope and the Spectro-Polarimetric High-contrast Exoplanet Research (SPHERE) instrument at the Very Large Telescope. Click here to view the video about the research. For more details, please refer to the journal paper ‘A retrograde planet in a tight binary star system with a white dwarf’, published in Nature.
NEWS DETAIL