16 Jun 2021
HKU first-year physics PhD student obtained the Higgs mode via dimensional crossover in quantum magnets which reveals importance of dimensions in many-body systems
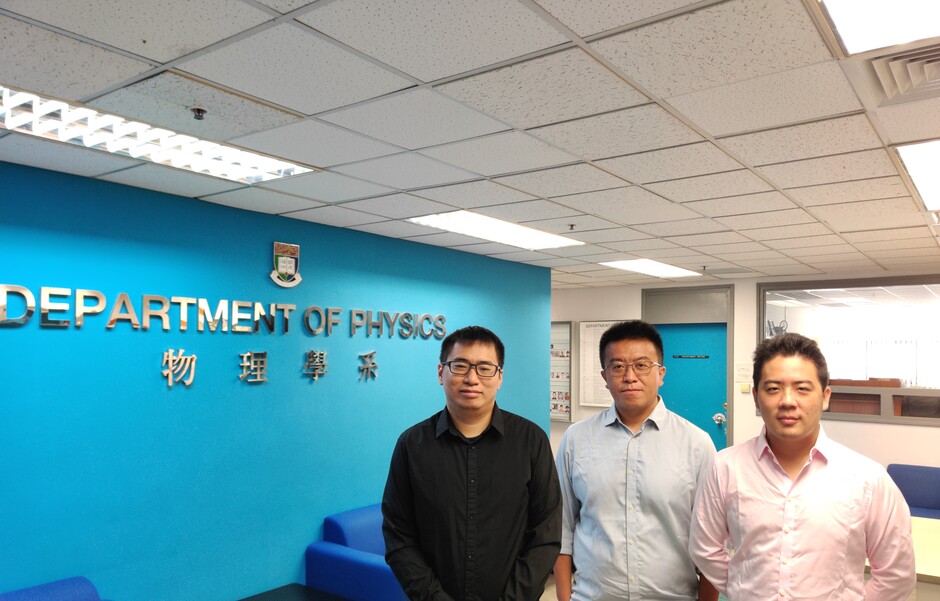
From the left: Mr Chengkang ZHOU, Dr Zi Yang MENG and Dr Zheng YAN from the Research Division for Physics and Astronomy, HKU Science.
In 2013, François ENGLERT and Peter HIGGS won the Nobel Prize in Physics for the theoretical discovery of a mechanism that contributes to our understanding of the origin of mass of subatomic particles, which was confirmed through the discovery of the predicted fundamental particle by the A Toroidal LHC Apparatus (ATLAS) and the Compact Muon Solenoid (CMS) experiments at The European Organization for Nuclear Research (CERN)'s Large Hadron Collider in 2012. The Higgs mode or the Anderson-Higgs mechanism (named after another Nobel Laureate Philip W ANDERSON), has widespread influence in our current understanding of the physical law for mass ranging from particle physics - the elusive “God particle” Higgs boson discovered in 2012 to the more familiar and important phenomena of superconductors and magnets in condensed matter physics and quantum material research.
The Higgs mode, together with the Goldstone mode, is caused by the spontaneous breaking of continuous symmetries in the various quantum material systems. However, different from the Goldstone mode, which has been widely observed via neutron scattering and nuclear magnetic resonance spectroscopies in quantum magnets or superconductors, the observation of the Higgs mode in the material is much more challenging due to its usual overdamping, which is also the property in its particle physics cousin – the elusive Higgs boson. In order to weaken these damping, two paths have been suggested from the theoretical side, through (1) quantum critical points and (2) dimensional crossover from high dimensions to lower ones. For (1), people have achieved several remarkable results, whereas there are few successes in (2).
To fulfil this knowledge gap, from 2020 Mr Chengkang ZHOU, then a first-year PhD student, Dr Zheng YAN and Dr Zi Yang MENG from the Research Division for Physics and Astronomy of The University of Hong Kong(HKU), designed a dimensional crossover setting via coupled spin chains. They applied the quantum Monte Carlo (QMC) simulation to investigate the excitation spectra of the problem. Teamed up Dr Hanqing WU from the Sun Yat-Sen University, Professor Kai SUN from the University of Michigan, and Professor Oleg A STARYKH from The University of Utah, they observed three different kinds of collective excitation in the quasi-1D limit, including the Goldstone mode, the Higgs mode and the scalar mode. By combining numerical and analytic analyses, they successfully explained these excitations, and in particular, revealed the clear presence of the Higgs mode in the quasi-1D quantum magnetic systems. All these results not only can help us find out the key model parameters of the material but also show us a picture of how dimension matters in the condensed material. These research finding has recently been published in one of the most prestigious journals in the field of physics – Physical Review Letters.
Background
The quantum materials have been embedded in our daily life, such as various electronic components, computer chips, and solar panels. With the fast development of science and technology, the understanding and manipulation of the quantum many-body interactions in the materials are playing a more and more important role. Such a tendency has already shown its first sign. For example, the 2D quantum moiré materials, such as the magic angle twisted bilayer graphene, have attracted much attention and show their novel performance in realising superconductivity in Carbon-based instead of the traditional silicon-based materials. Moreover, quantum computer tech, which is based on the theory of superconductivity and even topology is rapidly developing to build more efficient computer chips beyond Moore’s law. Along with these efforts, the research on quantum magnets is one of the most important objects, in which the detection of the Higgs mode and the Goldstone modes can reveal the underlying model parameters of the material.
However, since the interaction of billions of electrons must be considered, it is hard to point out a clear picture of several quantum many-body systems via quantum mechanics directly. Therefore, numerical methods, such as the QMC simulation, have become useful techs to study quantum many-body systems. These numerical methods can tell us useful information and properties of quantum many-body systems and show the micro mechanism of these systems. This information would stimulate the development of the theory as well as guiding the experimental design, which would help the scientists and engineers to find out more novel quantum materials and components.
The Higgs mode via dimensional crossover
To study the micro mechanism in quantum many-body systems, numerical physicists usually come up with a simple model based on theoretical understanding and experimental data. Then, they apply numerical simulation methods to study the model in reasonable parameters region. Such a research procedure has been used in the investigation of the Higgs mode in the quantum magnets, yet its observation is still challenging due to its usual overdamping feature. From the quantum-theoretical side, scientists have suggested two paths. The first is through the quantum critical point. On this path, there are several remarkable results, including the signal of the Higgs mode has been observed in . But the second path, which is though the dimensional crossover towards 1D, is still full of blank and calling for more study, partly because it is hard to find out a quantum many-body system with dimensional reduction. Such a dimensional reduction weakens the long-range order of the system and, therefore, inhibits the Higgs mode’s overdamping feature. The research team from The University of Hong Kong, the Sun Yat-Sen University, the University of Michigan and The University of Utah managed to fulfil these blanks by numerically simulating a dimensional-crossover quantum spin model, coupled spin chains (see Figure 1).
Image 1. Coupled spin chains with nearest-neighbour spin intrachain interaction (solid black line) and interchain interaction (red dashed line).
The research team came up with coupled spin chains model by introducing the interchain interaction. By reducing the strength of these interchain interactions (changing the value of in Figure 1 towards 0), the model would change from a 2D system to a quasi-1D system. The research team used the QMC method simulating the model and developed an effective method to measure the spin- and the bond- correlation functions. They not only observed the Higgs mode via a dimensional crossover but also find the scalar mode, which is predicted by the sine-Gordon theory. These results make coupled spin chains an attractive candidate system for studying collective quantum dynamics theoretically and experimentally.
The Higgs mode and the scalar mode spectrum
With the help of the Tianhe- II and III supercomputers, the research team study the coupled spin chains in the quasi-1D limit and observed the evolution of the spectrum of Goldstone mode(Figure 2 (g) and (k)), the Higgs mode(Figure 2 (h) and (l)), and the scalar mode(Figure 2 (f) and (j)). Figure 2 shows the obtained spectra from the QMC simulations, where the blue dashed line represents the dispersion relation according to the combination of the mean-field theory and the sin-Gordon theory model. As one can see in Figure 2, the numerical results fit well with the theory prediction, which means the research team has managed to catch the signal of the Higgs mode. These signal is very helpful to design corresponding experiments to observe the Higgs mode via a dimensional crossover, like neutron scattering and nuclear magnetic resonance spectroscopy. Such exciting results also will contribute to our understanding of the Higgs mode in dimensional reduction.
Image 2. The spectra of the Goldstone mode, the Higgs mode, and the scalar mode changes with the coupled spin chains that tends to a quasi-1D limit. The first and the third columns are for the Goldstone mode. The second tells the scalar mode while the fourth is the Higgs mode.
Image 3. Frequency dependence of the spectrum of the Higgs mode (b) and the scalar mode (a) evolve with the dimension reduction. In this dimensional reduction, a sharp peak is emerging, which means the signal of the scalar mode and the Higgs mode becomes stronger.
In order to describe the emergence of the Higgs mode and scalar mode, the research team also plotted out the frequency dependence of them (see Figure 3), where g is a factor telling how close the model to the quasi-1D is. When g=1, the coupled spin chains are in a 2D system, and with g=0, the coupled spin chains are in a 1D system. By comparing the frequency dependence of the spectrum with different g, one can find a sharp peak emerging as g reduces, which means the signals of the Higgs mode and the scalar mode are becoming stronger and stronger.
The novel physics phenomenon via dimensional crossover
In the framework of modern physics, symmetry and dimension are two of the most important factors that determine the properties of quantum many-body physics. And the phenomenon caused by dimension reduction is a key topic in quantum magnetic systems. The finding of the research team provided an attractive model and data support, which help us to understand what an essential role that the dimension plays in our world and stimulate the development of the next-generation quantum material and components.
About the team
Mr Chengkang Zhou is a PhD student in physics, under the supervision of Dr Zi Yang Meng.
The work was supported by the Research Grants Council of HKSAR, the Areas of Excellence “2D Materials Research: Fundamentals Towards Emerging Technologies”, and we thank the Computational Initiative at the Faculty of Science and the Information Technology Services at The University of Hong Kong and the National Supercomputer Centers in Guangzhou and Tianjin for their technical support and providing generous HPC resources that have contributed to the research results reported within this paper.
The journal paper can be accessed from here.