26 Jan 2023
HKU Chemist and collaborators engineered an effective and stable singe-atom catalyst which is a hundred times more effective than the commercial catalyst
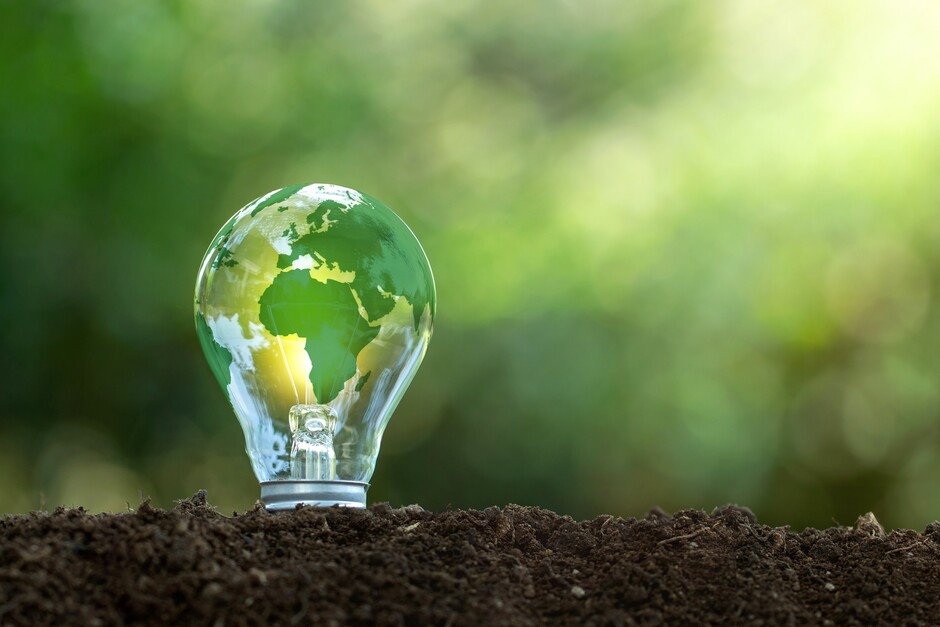
This study opens up a new avenue for developing SACs via effective electronic tuning of Pt single atoms with heterogeneous atomic coordination in hydroxide substrates.
Developing a cost-effective electrocatalyst for green hydrogen generation from water is crucial for the development of renewable energy technologies to resolve the current energy and environmental crisis. In a concerted research effort, Professor Zhengxiao GUO from the Department of Chemistry at The University of Hong Kong (HKU) and his long-term collaborators in mainland China, have engineered an effective and stable ‘single-atom catalyst’ (SAC), with Pt single atoms supported on a vacancy-enriched dual metal hydroxide, (Co,Ni)(OH)2. This catalyst exhibits a far superior hydrogen evolution reaction (HER) activity, which is 116 times higher than that of the commercial 20 wt% Pt/C catalyst, even with a small fraction of the Pt loading. There is also negligible attenuation or degradation after the electrochemical test at 100 mAcm−2 for 24 hours or cyclic voltammetry for 20000 cycles, indicating the high stability of the catalyst, even at a relatively high current density for potential practical applications. The findings have just been published in Energy and Environmental Science.
A sustainable clean energy supply is one of the foremost challenges to achieving ‘net-zero’ or carbon neutrality. Hydrogen is a promising clean energy carrier for such a purpose. However, its conventional production by methane reforming is accompanied by the generation of CO2 and also insufficient purity for the long-term operation of fuel cells. Using renewable power sources, such as solar or wind, is environmentally friendly for electrochemical water splitting to produce high-purity H2, which is increasingly important as the demand for hydrogen is likely to increase by 6-8 folds by 2050. However, this will create even more pressure on the already scarce Pt catalyst and its reserve. Moreover, the conventional cathode shows a relatively large overpotential and hence low efficiency (~60-80%) for H2 evolution, especially under alkaline conditions. Therefore, it is very desirable but challenging to develop a catalytic cathode that can efficiently evolve hydrogen at a relatively low overpotential under alkaline conditions. Despite considerable effort in the past, transition metal (TM) based catalysts usually show a relatively high overpotential and inferior catalytic performance to the commercial 20 wt% Pt/C, thus unfavourable for industrial applications.
To date, Pt is still the most active candidate due to the moderate free energy for the chemisorption of atomic hydrogen, which is more advantageous than other metals for the formation of H2 via the intermediate Pt-*H (* denotes 'adsorbed'). Unfortunately, the HER efficiency depends not only on ΔGH but also on the dissociation of water to obtain *H and *OH and the subsequent transfer of the dissociated species. The direct water dissociation on Pt to form Pt-*H is usually sluggish under an alkaline condition, due to a relatively high water-dissociation barrier. Compared to the Pt nanoparticles and clusters, Pt Single-Atom Catalysts (SACs) show readily regulatable electronic properties and high atom utilisation efficiency (or atomic economy) for various reactions. Such single atoms can be stabilised and anchored on another material or substrate, such as TM oxides and hydroxides, to impart further the electronic synergy for catalytic performance. Particularly, TM hydroxides (e.g., TM = Fe, Ni, Co) are characterised by low-cost, good alkaline stability, high hydrophilicity and distinctive electronic structures with partially-filled d orbitals to facilitate interactions with charge carriers. However, the pristine TM hydroxides still exhibit low efficiency for the HER due to their relatively high adsorption energies of *H and/or *OH after water dissociation, unfavourable for *H diffusion to the Pt site and/or continued water adsorption due to *OH accumulation, respectively.
In the combined electronic structural simulations and experimental analysis, the research team has identified that the bimetallic hydroxide of cobalt and nickel creates the electronic synergy for water dissociation with the 'just right' binding energies of *H and *OH to be transferred away from the hydroxide surface. Concurrently, the finely-tuned electronic microenvironment of Pt single atoms co-located over the (Ni,Co)(OH)2 substrate effectively reduced the energy barrier for hydrogen transfer on the (Ni,Co)(OH)2, to enhance hydrogen evolution. The generation of Ni-*OH and Co-*OH was directly observed by Operando Raman, indicating the enhancement of the (Co,Ni)(OH)2 for water dissociation in an alkaline electrolyte. Partial charge transfer from Pt to O atoms of (Co,Ni)(OH)2 creates an adequate local environment around the Pt, to attract the adsorbed *H species and then allow them to form hydrogen molecules with ease.
In summary, this study opens up a new avenue for developing SACs via effective electronic tuning of Pt single atoms with heterogeneous atomic coordination in hydroxide substrates. More specifically, the catalyst demonstrated great potential to break the bottleneck of low efficiency for hydrogen generation in alkaline fuel cells, currently under popular consideration in large-scale electrolysis of water to produce clean fuels of the future.
The journal paper can be accessed from here.